Killing cancer

Cancer is one of the most devastating diagnoses that a person can receive, and it is a society-wide problem. According to the National Cancer Institute, an estimated 2,001,140 new cases of cancer will be diagnosed in the United States and 611,720 people will die from the disease this year. While cancer treatments have seen large improvements over the last decades, researchers are still laser-focused on developing strategies to defeat cancers, especially those that have been resistant to traditional interventions.
At NYU Tandon, we’ve been taking cancer head on, with newly developed tracking and treatments aimed at battling this pernicious ill.
Lab-on-a-chip
B cell acute lymphoblastic leukemia (B-ALL) hijacks the bone marrow (BM) microenvironment to form leukemic BM “niches,” facilitating chemoresistance and, ultimately, disease relapse. The key to developing more effective, targeted therapies depends on researchers' ability to isolate and examine with these evolving, heterogeneous interactions among distinct B-ALL subtypes and their varying BM niches. Current in vivo methods limit that ability.
A team led by Weiqiang Chen, Associate Professor of Biomedical and Mechanical and Aerospace Engineering, demonstrated an in vitro organotypic “leukemia-on-a-chip” model to emulate the in vivo B-ALL BM pathology and comparatively studied the spatial and genetic heterogeneity of the BM niche in regulating B-ALL chemotherapy resistance.
In the study "Leukemia-on-a-chip: Dissecting the chemoresistance mechanisms in B cell acute lymphoblastic leukemia bone marrow niche," published in ScienceAdvances, the team used their system to describe the heterogeneous chemoresistance mechanisms across various B-ALL cell lines and patient-derived samples, showing that the leukemic perivascular, endosteal, and hematopoietic niche-derived factors maintain B-ALL survival and quiescence. Furthermore, they demonstrated the preclinical use of their lab-on-a-chip model to test niche-cotargeting regimens, which may translate to patient-specific therapy screening and response prediction.
A unique way to test brain cancer treatments
The lab-on-a-chip is not the only way Tandon engineers are building unique testing for cancer treatments. Nikita Grigoryev is immersed in the study of glioblastoma, the deadliest form of brain cancer. Glioblastoma tumors can be excised, but the aggressive cells that escape the scalpel end up killing the vast majority of patients within five years of diagnosis. Previously, there was no effective way to study the spread of glioblastoma cells; in vivo experiments involving live animals came with a set of ethical dilemmas, and the brains of mice do not, at any rate, exactly mimic those of humans.
Grigoryev has engineering an artificial scaffolding that enables cells from glioblastoma patients to grow in the lab to be studied. The process of building such scaffolding consists of electrospun nanofibers that are embedded in a clear hydrogel, closely mimicking the cells’ natural environment. Because the hydrogel is transparent, researchers can easily distinguish between the original cells and the newly grown cells, tracking them, studying their gene amplification, and giving new hope to patients.
New scans for breast cancer
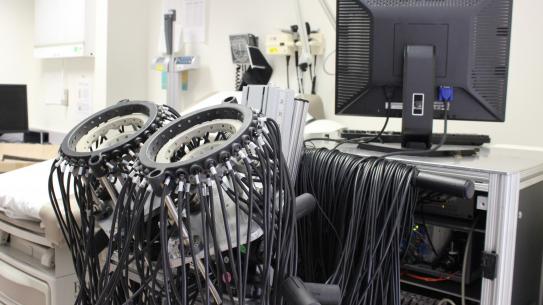
In the United States, roughly 13% of women will develop breast cancer, and more than 43,000 women are expected to die as a result in 2021. The disease represents a serious problem for public health. And while screening for the disease can help prevent catastrophic results, monitoring the progression of cancer and how it responds to treatment can be difficult.
Now researchers at NYU Tandon are developing the technology to help track the development of breast cancer, without causing further harm. Andreas Hielscher oversees the Clinical Biophotonics Laboratory at Tandon, where he focuses on developing new medical imaging technologies that use near-infrared light instead of more common X-rays or ultrasound. Light transmitted through the human body, he has explained, can provide information about the blood and oxygen supply in various tissues, and that information can be used by clinicians to diagnose and monitor various diseases–including breast cancer, rheumatoid arthritis, and peripheral artery disease (PAD) in diabetic patients.
Using a new ceramic sensor technology developed to detect subtle variations in breast tissue, Matthew Campisi (’96, ‘20) developed iBreastExam, a battery-operated wireless machine that records variations in breast elasticity. It’s hand-held and allows health care workers to perform breast examinations in five minutes, anywhere. Painless and radiation-free, it provides results just a few minutes after the exam through a mobile app, which also records patients’ data. that the device demonstrated excellent sensitivity, meaning it was able to correctly detect clinically significant lesions in patients. The invention earned Campisi the distinction of 2019 NYU Alumni Changemaker.
Proteins engineered to join the fight
In the ongoing quest to develop more effective cancer therapies, Jin Kim Montclare has unveiled a promising approach utilizing protein-based targeting agents. A recent study published in Biomaterials Science introduces a novel strategy that harnesses the power of multivalent assembled proteins (MAPs) to target hypoxic tumors with unprecedented precision and efficacy.
Traditional cancer treatments often rely on passive or active targeting mechanisms to deliver therapeutic agents to tumor sites. However, these approaches have limitations, particularly in overcoming physiological and pathological barriers. To address these challenges, the research team focused on exploiting the unique features of the tumor microenvironment (TME), specifically its hypoxic conditions.
Hypoxia, a characteristic feature of many solid tumors, is known to play a crucial role in tumor progression and resistance to therapy. By targeting hypoxia-inducible factor 1 alpha (HIF1α), a key regulator of cellular response to low oxygen levels, the researchers aimed to develop a more effective strategy for tumor-specific drug delivery.
Previous efforts to target HIF1α have been hindered by the instability and limited binding abilities of the peptide-based molecules used. To overcome these obstacles, the team turned to MAPs, which offer the advantages of high stability and multivalency.
Drawing inspiration from their successful development of MAPs targeting COVID-19, the researchers engineered HIF1α-MAPs (H-MAPs) by grafting critical residues of HIF1α onto the MAP scaffold. This innovative design resulted in H-MAPs with picomolar binding affinities, significantly surpassing previous approaches. In vivo studies showed promising results, with H-MAPs effectively homing in on hypoxic tumors.
Montclare’s findings suggest that H-MAPs hold great potential as targeted therapeutic agents for cancer treatment. With further refinement and exploration, H-MAPs could offer a new avenue for precision medicine, providing clinicians with a powerful tool to combat cancer while minimizing side effects and maximizing therapeutic efficacy.